光纤锁模激光器:从单模时域耗散孤子锁模到多模时空耗散孤子锁模(特邀)
下载: 642次创刊五十周年特邀【增强内容出版】
在光纤锁模激光器中,模式相位锁定产生周期短脉冲的过程称为锁模过程,产生的脉冲在广义上被称为“光耗散孤子”。光纤锁模激光器从传统的单模光纤锁模激光器发展到了多模光纤锁模激光器,锁模机理从一维(1D)时域耗散孤子锁模发展到了(3+1)维时空耗散孤子锁模。通过深入理解耗散孤子的产生机理,有望进一步推动光纤锁模激光器在科学和应用领域的发展,为更多领域带来更多创新和可能性。首先介绍单模光纤锁模激光器中的一维时域耗散孤子锁模,探讨不同色散区域中时域耗散孤子的产生机理;随后介绍多模光纤锁模激光器中时空耗散孤子的最新研究成果,讨论模间色散的补偿方法,揭示其丰富的时空锁模机理和潜在的应用场景;最后对光纤锁模激光器的发展前景进行展望。
Fiber-mode-locked lasers play an important role in generating ultrashort pulses of picosecond or even femtosecond durations, featuring high peak power and broad spectral characteristics. These pulses have important applications in precision machining, spectroscopic measurements, high-capacity optical communications, terahertz technology, and nonlinear optical imaging. Ultrashort pulses generated by fiber-mode-locked lasers result from a double balance between dispersion and nonlinearity, as well as between gain and loss. Grelu et al. extended the concept of dissipative solitons to include ultrashort pulses generated in nonconservative systems such as fiber-mode-locked lasers. These stable dissipative solitons exist in a continuous exchange of energy with the environment and a dynamic energy redistribution between the components of the soliton. In a mode-locked fiber laser, the process of mode phase-locking to produce periodic short pulses is known as mode locking, and the resulting pulses are generally termed optical dissipation solitons. A comprehensive understanding of the mechanisms underlying dissipative soliton generation holds great promise for advancing mode-locked fiber lasers in both scientific and practical applications, offering greater innovation and possibilities across a wider range of fields.
The peak pulse power generated by single-mode fiber mode-locked lasers approaches its limitations in the megawatt (MW) order, which are limited by the number of modes and the mode-field area of the single-mode fibers. To further enhance the performance of mode-locked fiber lasers, it is essential to consider higher dimensions (i.e., introducing spatial dimensions) and explore the impact of increasing the spatial modes (i.e., transverse modes) on soliton mode-locking in multimode fiber lasers. Consequently, mode-locked fiber lasers have evolved from traditional single-mode to multi-mode configurations, and mode-locking mechanisms have transitioned from one-dimensional (1D) temporal dissipative soliton mode-locking to (3+1)D spatiotemporal dissipative soliton mode-locking. The expansion of spatial dimensions results in complex nonlinear spatiotemporal interactions and rich physical spatiotemporal phenomena. Spatiotemporal dissipative solitons not only exhibit periodic pulse output in the time domain but also show the distribution characteristics of multiple transverse modes in the space domain. Spatiotemporal dissipative solitons achieved using multimode fiber lasers have potential applications in precision ranging, laser processing, nonlinear spectroscopy, optical tweezers, and scattering medium imaging, offering new possibilities in information transmission and imaging.
In this review, we focus on the study of dissipative soliton generation mechanisms in fiber mode-locked lasers, trace the development of fiber mode-locked lasers, and review the principles of generating one-dimensional temporal dissipative solitons in single-mode fiber lasers to generate three-dimensional spatiotemporal dissipative solitons in multimode fiber lasers. First, we explore the generation mechanisms of temporally dissipative solitons in single-mode fiber lasers with different chromatic dispersions. Temporal dissipative solitons can form when the positive chirp (owing to self-phase modulation) balances the negative chirp (owing to an anomalous even-order dispersion). Early studies generally considered the case in which self-phase modulation balances second-order dispersion, resulting in the formation of a second-order dispersion soliton (Fig.2). Higher-order even-order dispersive solitons can also be levelled with self-phase modulation and form the corresponding solitons, which are referred to as higher-even-order dispersive solitons (Fig.3). Notably, stable temporally dissipative solitons can be generated even in the absence of dispersion, and this type of soliton is referred to as a dispersionless soliton (Fig.4). The different types of solitons have different properties, and their formation involves various physical processes.
Subsequently, we delved into the latest achievements in spatiotemporal dissipative soliton mode-locking in multimode fiber lasers. In contrast to temporal dissipative solitons in single-mode fiber lasers, spatiotemporal dissipative solitons in multimode fiber lasers add spatial dimensions by incorporating multiple transverse modes. In this case, the dispersion consists of both chromatic (intramode) and intermode (modal) dispersions. Therefore, balancing the modal dispersion is important to generate spatiotemporal dissipative solitons in a multimode fiber laser. We discuss compensation methods for modal dispersion (Fig.5) and reveal their rich spatiotemporal mode-locking mechanisms and potential application scenarios. Finally, we provide an outlook on the research prospects for mode-locked fiber lasers.
In this paper, we present the research history from the traditional single-mode temporal dissipative soliton to a more complex multimode spatiotemporal dissipative soliton and summarize the generation mechanisms of various dissipative solitons in fiber lasers. Through this development, we aim to summarize the differences between temporal and spatiotemporal dissipative solitons, emphasize the significance of understanding the mode-locking mechanism for conducting research and the application of fiber-mode-locked lasers, and show the potential application scenarios of fiber-mode-locked lasers in the future. In summary, focusing on temporal/spatiotemporal dissipative solitons in fiber lasers not only enhances our understanding of the principle of fiber mode-locking lasers but also opens up more opportunities for fiber laser applications. With the continuous progress of technology and theoretical improvements, we believe that fiber-mode-locked lasers will continue to play an important role in the future and provide more possibilities for applications in optical frequency combs, material processing, medical diagnostics, and other fields.
1 引言
光纤锁模激光器在现代光学领域扮演着重要角色,它可以产生持续时间在皮秒乃至飞秒量级的超短脉冲,具有高峰值功率和宽光谱的特性,在微纳精密加工、精密光谱测量、大容量光通信、太赫兹技术和非线性光学成像等领域具有重要应用[1-4]。在有源介质(通常为掺杂光纤)提供增益的条件下,光纤激光器中不同频率的光可以同时振荡,这些不同频率的光被称为“纵模”。当各纵模之间存在确定的相位关系时,时域上就会周期性地叠加产生超短脉冲。在光纤激光器中,这种通过模式相位锁定产生周期短脉冲的过程被称为“锁模”。由于系统的非保守特性,产生的脉冲在广义上也被称为“光耗散孤子”。
1973年,Hasegawa等[5-6]在理论上证明了无源光纤中孤子的存在,并认为可将其用于光纤通信。在他们的理论中,光纤中的孤子传输可以用非线性薛定谔方程来描述:当光纤中的群速度色散为负数(即群速度色散系数β2<0,也称为“反常色散”)时,光纤支持亮孤子;当光纤中的群速度色散为正数时,光纤支持暗孤子。1980年,贝尔实验室的Mollenauer等[7]率先实现了光纤孤子。1984年,Mollenauer和Stolen通过将一段保偏光纤引入光纤激光器实现了孤子输出,他们将这种光纤激光器称为“孤子激光器”。之后,Haus等[8]建立了孤子激光器的解析理论。相较于光纤中传播的孤子,光纤激光器中光孤子的形成是腔色散、光纤非线性、激光增益饱和、可饱和吸收效应和增益带宽滤波等因素相互作用的结果。对此,Haus等[9]认为可以使用主方程来描述锁模激光谐振腔中光脉冲的演化,研究结果显示该方法适用于大部分锁模系统。主方程也被称为“三次金兹伯格朗道方程”(CGLE),其以分布的方式处理腔内的所有效应,描述了激光器中的平均脉冲传播。但该方程的解只在较小的增益参数范围内是稳定的。在其基础上加入五次项增益得到的三次-五次金兹伯格朗道方程(CQGLE)可以更好地描述孤子光纤锁模激光器[10]。至此,孤子光纤锁模激光器的理论模型成功建立起来。1994年,Vanin等[11]发现在具有宽带增益和群速度色散的非线性光学介质中可以存在耗散型的稳定孤子结构,并将其称为“耗散孤子”。光纤锁模激光器产生的超短脉冲是色散和非线性以及增益和损耗之间双重平衡的结果,因此,Grelu等[12]引申了传统的保守孤子的概念,将光纤锁模激光器这种非保守系统中产生的超短脉冲称为“耗散孤子”,即稳定的耗散孤子与环境之间存在持续的能量交换,并且耗散孤子各部分之间存在动态的能量再分配。
为了更好地理解光纤锁模激光器中的耗散孤子,需要细致地考察传统(保守)孤子和耗散孤子之间的差异。关于光纤锁模激光器中耗散孤子的定义与理解,目前存在两种理解。一种理解是:激光腔中通过反常色散和自相位调制平衡产生的脉冲为传统孤子,而通过色散与耗散效应(例如增益带宽、光谱滤波等)平衡产生的脉冲为耗散孤子[11]。在这种情况下,并不是所有的光纤锁模激光器产生的脉冲都是耗散孤子。另一种理解是:哈密顿保守系统中产生的孤子为传统孤子,而非保守系统中产生的孤子为耗散孤子。耗散孤子是增益与损耗以及色散与非线性之间双重平衡的结果[12-13]。近日,Grelu[14]采用这种理解扩展了耗散孤子的定义。在锁模激光器中,只有适当考虑耗散效应,才能确定稳定脉冲动力学的关键特征。这种看法认为,所有的光纤锁模激光器都是非保守系统,故其产生的锁模激光脉冲可以统一称为“耗散孤子”。本文采用Grelu对耗散孤子的理解,将单模光纤锁模激光器中产生的锁模脉冲统一称为“时域耗散孤子”,将时空锁模光纤激光器中产生的锁模脉冲称为“时空耗散孤子”。
在单模光纤激光器中,当各纵模之间存在确定的相位关系时,各纵模在时域上叠加就会产生周期性超短脉冲,即产生时域耗散孤子。但由于单模光纤的芯径小且模场面积有限,由其构成的单模光纤锁模激光器产生的脉冲峰值功率在兆瓦(MW)量级[15],已经接近其极限。如何进一步提升锁模激光器的性能,需要从更高的维度,即从空间模式入手,探究多模光纤激光器中空间模式(即横模)增加对孤子锁模的影响。这不仅能丰富孤子光纤锁模激光器的理论研究,还有利于提高脉冲能量,拓展光纤锁模激光器的应用范围。多模光纤中光孤子的研究已经持续多年,众多丰富的时空物理现象,如时空不稳定性[16-18]、克尔自清洁[19-21]、保守多模孤子[22-25]、模间四波混频[26-29]、非线性多模干涉[30]等,相继被发现。但由于模间色散的存在,在多模光纤构成的振荡器中实现耗散孤子(锁模)一直是一个挑战。早在1968年,Auston[31]就已经在理论上讨论了横模锁定的可能性,并在模式数很少的He-Ne激光腔中实现了纵模和横模的同时锁定[32]。30年后,Côté等[33]在钛蓝宝石飞秒激光器中观察到了两个横模的锁定。但是,在这些研究中参与锁定的横模数量很少。光纤激光器中多横模锁定的现象一直未实现,加之多模光纤激光器中的横模耦合过于复杂,故光纤激光腔中的时空锁模理论一直未建立。直到2017年,康奈尔大学Wise组的Wright等[34]才首次在多模光纤激光器中实现了多横模锁定,他们将这种光纤激光器命名为“时空锁模光纤激光器”。Wright等的这一工作促进了光纤激光器中时空锁模理论模型的建立。但是,目前光纤激光器的时空锁模理论研究依然处于发展阶段。空间维度的扩展带来了复杂的非线性时空相互作用和丰富的时空物理现象。类比于时域耗散孤子,这种具有特殊空间分布的耗散孤子被称为“时空耗散孤子”[35]。它不仅在时域上表现为周期性脉冲输出,还在空间上呈现出了多横模分布特性。多模光纤激光器实现的时空耗散孤子在精密测距、激光加工、非线性光谱学、光镊和散射介质成像等领域具有潜在的应用价值,为信息传输和成像等领域取得重大进展提供了新的可能途径[36-41]。通过深入研究时空耗散孤子的产生机理,可以更好地观察、理解高维非线性动力学过程,并有望进一步推动光纤锁模激光器在科学和应用领域的发展。
本综述将介绍传统的单模时域耗散孤子到更加复杂的多模时空耗散孤子的研究历程,总结光纤激光器中各种耗散孤子的产生机理。首先针对基于单模光纤的锁模激光器,讨论不同色散区域内时域耗散孤子的产生;随后探讨多模光纤锁模激光器中时空耗散孤子的实现办法,以模间色散的补偿为对象,讨论不同模间色散情况下时空耗散孤子的产生机理及其潜在的应用场景。笔者梳理光纤锁模激光器发展历程的主要目的是总结时域耗散孤子和时空耗散孤子的区别,展示锁模机理在开展光纤锁模激光器研究方面的重要性,并尝试展现光纤锁模激光器未来潜在的应用场景。
2 一维时域耗散孤子锁模
在光纤锁模激光器中,耗散孤子的形成是色散与非线性效应以及增益与损耗之间平衡的结果[12],如
式中:βm表示第
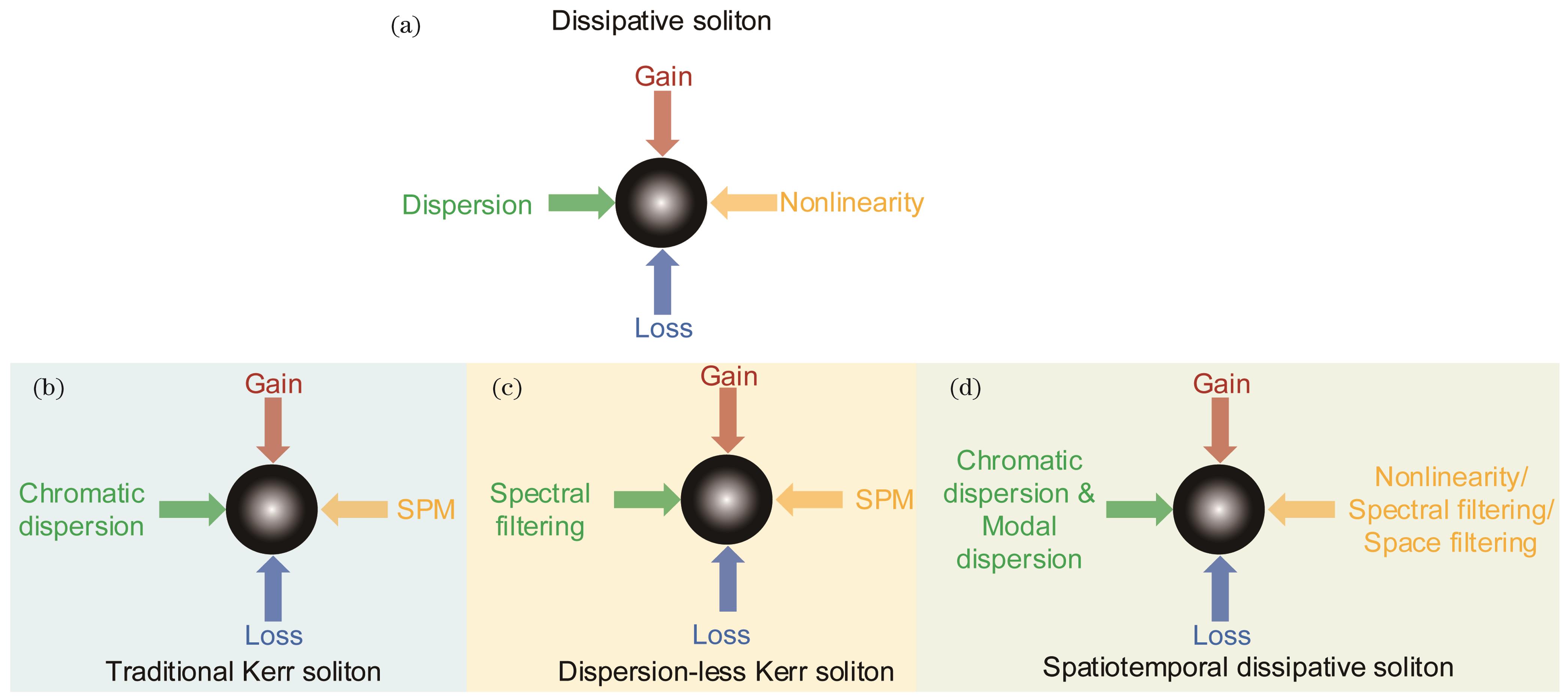
图 1. 光纤锁模激光器中耗散孤子的形成原理。(a)光纤锁模激光器中耗散孤子的形成依赖色散与非线性效应以及增益与损耗之间的平衡;(b)传统孤子的形成依赖于反常色散和自相位调制(SPM)的平衡;(c)无色散孤子的形成依赖于光谱滤波和自相位调制的平衡;(d)时空耗散孤子形成过程中的色散包括色度色散和模间色散,其可以由非线性、光谱滤波和空间滤波共同平衡
Fig. 1. Schematic diagram of dissipative soliton formation in fiber mode-locked lasers. (a) Dissipative soliton generation requires double balance between dispersion and nonlinearity as well as gain and loss; (b) the formation of conventional soliton relies on the balance between anomalous dispersion and self-phase modulation (SPM); (c) the formation of dispersion-less soliton relies on the balance between spectral filtering and self-phase modulation; (d) the dispersion in the process of formation of spatiotemporal dissipative soliton includes chromatic dispersion and intermode dispersion (steady propagation of spatiotemporal dissipative solitons in multi-mode fiber lasers needs delicate balance between gain, nonlinear loss, linear loss, nonlinearity and dispersion)
2.1 二阶色散孤子
二阶色散孤子的形成和传播依赖于自相位调制和二阶色散的平衡,见
2.1.1 反常色散孤子
早期的理论表明,通过解析非线性薛定谔方程,反常色散光纤中的自相位调制和二阶色散可以在腔内平衡,从而产生稳定的孤子解[43]。反常色散光纤激光器中的孤子首次出现于20世纪90年代。这种孤子的典型形状为双曲正割形[44-45],目前普遍被称为“传统孤子”(本文称之为“反常二阶色散孤子”),产生这种孤子的光纤激光器通常被称为“孤子光纤激光器”。在这种情况下,激光腔内的净色散为负值,会导致脉冲产生负啁啾;与此同时,自相位调制导致脉冲产生正啁啾。这两种效应达到平衡后,脉冲便以无啁啾孤子的形式传输,如
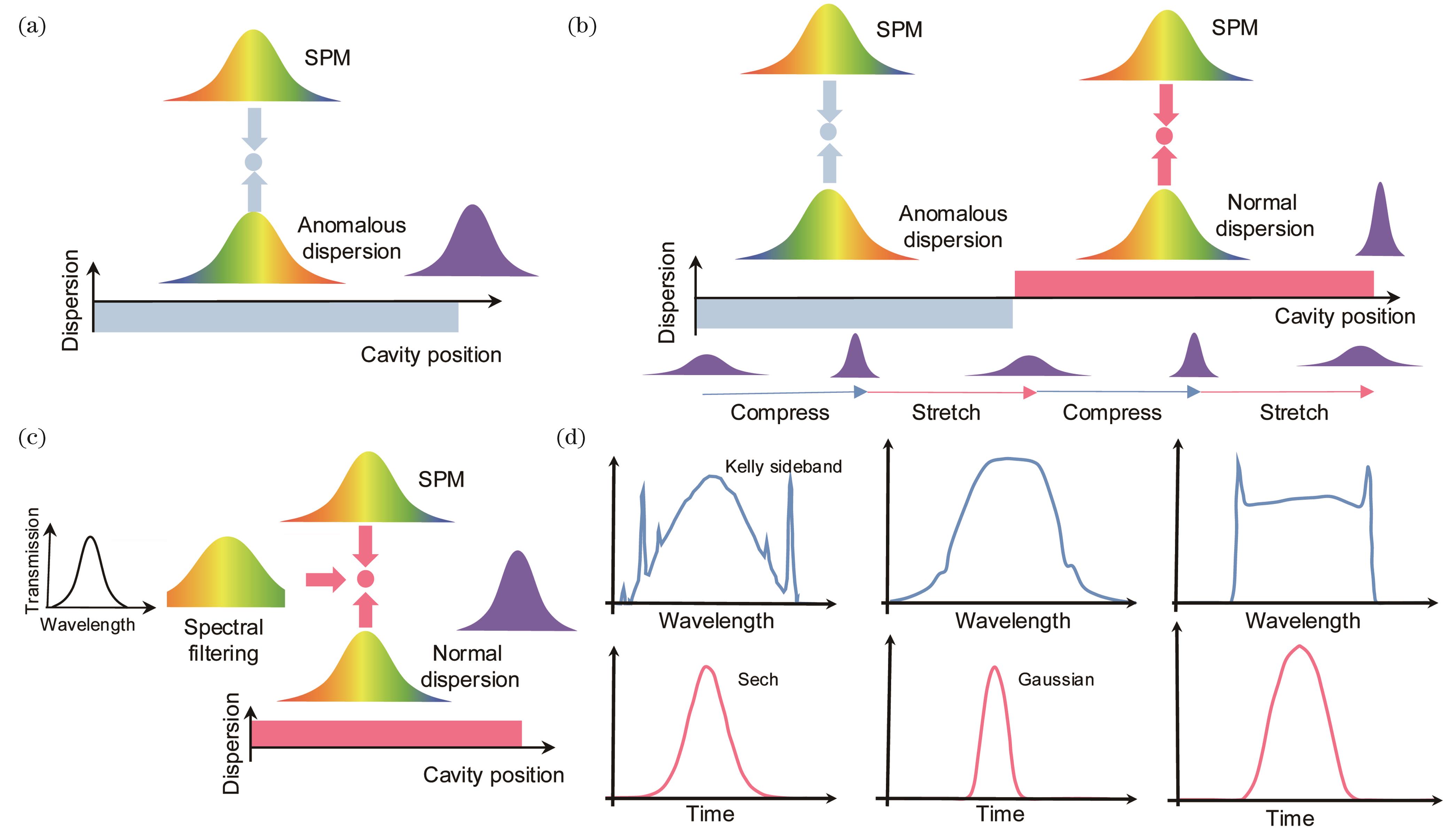
图 2. 光纤锁模激光器中二阶色散孤子的产生原理图。(a)反常色散光纤中的自相位调制(SPM)和色散可以在腔内平衡,从而产生稳定的孤子;(b)正常色散和反常色散都存在,在色散与非线性共同作用下,光脉冲经历周期性的展宽和压缩,形成近零色散孤子;(c)在全正常色散激光腔中,正常色散和非线性都会导致脉冲展宽,通过加入光谱滤波器来减小脉宽的展宽,从而保证光脉冲传输的自洽;(d)反常色散孤子(左)、近零色散孤子(中)和全正常色散孤子(右)的光谱和时域脉冲
Fig. 2. Schematic diagram of second-order dispersive soliton formation in fiber mode-locked lasers. (a) Self-phase modulation (SPM) and anomalous dispersion can be balanced in the cavity, resulting in stable solitons; (b) both normal and anomalous dispersion exist, and the pulse undergoes periodic broadening and compression by the combined effect of dispersion and nonlinearity, forming near-zero dispersion solitons; (c) in an all-normal-dispersion laser cavity, both normal dispersion and nonlinearity result in pulse broadening, and pulse width broadening is minimized by the addition of a spectral filter to reduce the pulse broadening, thus ensuring the self-consistency of the pulse transmission; (d) optical spectra and pulse trains of anomalous dispersive soliton (left), near-zero dispersive soliton (middle), and all-normal dispersive soliton (right)
2.1.2 近零色散孤子
为了进一步提高光纤锁模激光器的输出能量,引入了近零色散孤子的概念。这一概念最早由Fermann等[52]提出。他们设计了一种展宽脉冲光纤激光器,该激光器也被称为色散管理光纤激光器。这种激光器结合了正常色散和反常色散元件(这里所说的色散都是二阶色散),可使腔内的色散接近于零或微负值。在这种情况下,孤子锁模原理也有所不同。如
2.1.3 全正常色散孤子
当在近零色散孤子腔内增加泵浦功率时,仍然可能出现脉冲分裂。然而,通过在腔内引入一定的正常色散,可以扩大脉冲宽度、降低脉冲峰值功率,从而减少脉冲经历的非线性效应,有效地避免脉冲分裂问题。事实上,在正常色散条件下也存在孤子脉冲解,这一理论由Bélanger等[55]在1989年首次证明。直到2006年,康奈尔大学的Chong等[56]通过在激光腔内引入光谱滤波器,首次实现了全正常色散光纤锁模激光器,其单脉冲输出能量达到了3 nJ,该锁模激光器被简称为“ANDi光纤激光器”。
在全正常色散条件下,脉冲传输具有极高的正啁啾,输出脉宽通常为皮秒级。皮秒级脉冲能有效降低脉冲峰值功率,减弱非线性相移的累积,从而抑制脉冲分裂现象。此外,如
此外,正常色散光纤激光器中也可以产生自相似脉冲。自相似脉冲的概念最早由Anderson等[59]在1993年提出。他们指出,具有线性啁啾的脉冲在正常色散光纤中传输时会经历自相似的演化过程,时域上为抛物线状的脉冲波形是非线性薛定谔方程的渐近解,脉冲形状保持不变。这种演化模式可以使脉冲在较大的非线性效应下不发生脉冲分裂,从而可以具有较高的脉冲能量[60]。2004年,Ilday等[61]将自相似脉冲的概念应用到光纤激光器中,提出了自相似光纤激光器,该激光器也被称为无波分裂激光器。在该激光器中,非线性效应与正常色散效应的共同作用使得脉冲脉宽单调展宽并积累正的啁啾,需要额外引入啁啾补偿或者光谱滤波机制,以便使腔内的自相似脉冲满足自洽条件。2010年,自相似-孤子激光器也被提出[62],其激光腔内同时存在自相似放大和孤子类型的脉冲,但仍然属于色散管理腔。同年,Renninger等[63]发现减小滤波器带宽可使脉冲很容易演化到自相似区域,并基于这一方法在ANDi腔内实现了自相似脉冲。相比一般ANDi腔产生的耗散孤子,这里的自相似脉冲的形状为抛物线,并且具有超过20的腔内光谱呼吸比,因此可以在使用小带宽滤波器时产生更高能量的脉冲,脉冲也更容易压缩到接近傅里叶变换极限。
总的来说,光纤锁模激光器中不同的二阶色散孤子类型为提高输出能量和控制脉冲性质提供了多种方法,每种类型的二阶色散孤子都有其独特的特点和应用潜力。在了解其不同的锁模原理后,通过合理设计光纤激光器的参数和引入适当的元件,可以实现不同类型的孤子,从而满足不同应用领域的需求。
2.2 高偶数阶色散孤子
传统上,光纤锁模激光器中耗散孤子的形成只考虑非线性效应与二阶色散之间的平衡。高阶色散往往被认为会对孤子激光器的性能产生不利影响,比如会导致色散波产生以及脉冲畸变等[64-65]。然而,近年来,国内外研究人员对具有高阶色散的激光器,特别是四阶色散激光器[66-68],表现出了极大的兴趣。这种激光器通常具有大的四阶色散、较小的二阶色散以及可忽略的三阶色散,耗散孤子脉冲仅在四阶反常色散与自相位调制效应平衡的情况下产生[69-70],如
![高偶数阶色散孤子。(a)四阶色散孤子形成的原理图。反常四阶色散会导致脉冲时域加宽,但光谱形状不变,而自相位调制在不干扰脉冲时域形状的情况下导致光谱加宽,两种效应共同作用可以保证四阶色散孤子产生[66]。(b)四阶色散孤子的光谱(左)和时域(右)表征,其中脉冲中心附近具有指数衰减的周期性振荡尾巴,而且脉冲中心呈现出近似高斯形状[67]。(c)高偶数阶色散孤子的光谱以及时域的线性坐标(左)和对数坐标(右)表征,其中蓝色、红色、绿色和黑色曲线分别表示数值计算得到的六阶、八阶、十阶和十六阶色散孤子的时域和光谱[42]](/richHtml/zgjg/2024/51/1/0101003/img_03.jpg)
图 3. 高偶数阶色散孤子。(a)四阶色散孤子形成的原理图。反常四阶色散会导致脉冲时域加宽,但光谱形状不变,而自相位调制在不干扰脉冲时域形状的情况下导致光谱加宽,两种效应共同作用可以保证四阶色散孤子产生[66]。(b)四阶色散孤子的光谱(左)和时域(右)表征,其中脉冲中心附近具有指数衰减的周期性振荡尾巴,而且脉冲中心呈现出近似高斯形状[67]。(c)高偶数阶色散孤子的光谱以及时域的线性坐标(左)和对数坐标(右)表征,其中蓝色、红色、绿色和黑色曲线分别表示数值计算得到的六阶、八阶、十阶和十六阶色散孤子的时域和光谱[42]
Fig. 3. Higher even-order dispersive solitons. (a) Schematic diagram of fourth-order dispersion soliton formation in fiber lasers. The anomalous fourth-order dispersion leads to a temporal broadening of the pulse but no change the spectral shape, while the self-phase modulation leads to a broadening of the spectrum without disturbing the pulse in time domain. The two effects together ensure that fourth-order dispersion solitons are produced [66]. (b) Spectrum (left) and pulse train (right) of a fourth-order dispersive soliton with an exponentially decaying periodic oscillatory tail near the center of the pulse, and the center of the pulse exhibits an approximately Gaussian shape[67]. (c) Spectra of high-even-order dispersive solitons and temopral characterization in linear (left) and logarithmic (right) coordinates, where blue, red, green, and black curves denote the time domain and spectra of numerically computed sixth-, eighth-, tenth-, and sixteenth-order dispersive solitons, respectively[42]
1994年,Karlsson等[71]首次预测了四阶反常色散条件下存在一种类似孤子脉冲的现象,并且观察到这些脉冲在传输过程中呈现前后振荡的特点。随后的研究逐渐揭示了四阶反常色散对脉冲窄化的影响。1996年,Piché等[72]通过理论分析证明了在四阶反常色散条件下存在稳定的孤子解析解。2013年,Roy等[73]发现只有在同时存在四阶反常色散和自相位调制效应的情况下,才能够实现脉冲的稳定传输,并提出了“四阶色散孤子”的概念。随着时间的推移,Blanco-Redondo等[66]于2016年通过特殊设计的光子晶体波导,首次在实验中成功实现了由四阶反常色散主导的四阶色散孤子的传输。2020年,Runge等[67]将纯四阶色散孤子的概念与光纤激光器相结合,通过在腔内加入光谱整形器来补偿二阶色散,在掺铒光纤激光器内实现了四阶孤子的传输。在四阶色散孤子腔内,四阶反常色散会导致脉冲的时域展宽,但光谱形状保持不变,而自相位调制则会导致光谱展宽,但不会影响脉冲形状。当这两种效应相互作用时,就可以实现四阶色散孤子的稳定产生与传输,如
与传统的双曲正割孤子不同,四阶色散孤子具有一些显著特征。首先,脉冲表现出呈指数衰减的周期性振荡尾巴,同时脉冲中心呈现出近高斯形状,这种形状特征可以在
随着对四阶色散孤子研究的深入,高阶偶数色散孤子的研究也开始逐渐增多。这类孤子可以在克尔非线性和高阶反常偶数色散存在的情况下产生。研究发现,高阶偶数色散孤子具有与四阶色散孤子类似的特性,包括周期性振荡尾部和平坦的光谱,这些特性可以在
2.3 无色散孤子
从上面的研究历程可知,色散一直被认为是光纤激光器中孤子形成的关键条件。然而,色散主导的孤子在脉冲和光谱(光梳)形状上一般是确定的,不易调控,不利于实际应用。举例来说,为了在光通信中应用,理想的光梳应该展现出各个梳齿功率均等的矩形光谱,然而,反常色散区的孤子光梳通常呈双曲正割形状,而正常色散区的孤子光梳则伴随着显著的功率波动,与理想形状相去甚远[74]。清华大学的薛晓晓、郑小平研究团队与法国勃艮第大学的Grelu教授团队[74]合作发现了一种以光谱滤波效应为主导的孤子产生机制,如
![无色散孤子[74]。(a)无色散孤子的物理机理。随着滤波阶数的增加,光谱滤波带来的损耗下降;当光谱滤波阶数趋于无穷大时,光谱滤波效应和自相位调制达到平衡,参量增益和均匀损耗达到平衡。(b)无色散孤子的时域(左)和光谱(右)](/richHtml/zgjg/2024/51/1/0101003/img_04.jpg)
图 4. 无色散孤子[74]。(a)无色散孤子的物理机理。随着滤波阶数的增加,光谱滤波带来的损耗下降;当光谱滤波阶数趋于无穷大时,光谱滤波效应和自相位调制达到平衡,参量增益和均匀损耗达到平衡。(b)无色散孤子的时域(左)和光谱(右)
Fig. 4. Dispersion-less solitons[74]. (a) Mechanism of the dispersion-less solitons. The loss due to spectral filtering decreases as the filtering order increases. When the spectral filtering order tends to infinity, the spectral filtering effect and self-phase modulation reach equilibrium, and the parametric gain and uniform loss reach equilibrium. (b) Temporal pulse train (left) and optical spectrum (right) of a dispersion-less soliton
3 三维时空耗散孤子锁模
与单模光纤锁模激光器中的时域耗散孤子相比,多模光纤锁模激光器中的耗散孤子在时域传输的基础上增加了空间维度(多横模)。在这种情况下,色散不仅包括色度色散(模内色散),还涉及模间色散。模间色散是指不同横模在多模光纤中的传播群速度不同导致横模在光纤中逐渐分散并走离。因此,在多模光纤激光器中产生耗散孤子时,不仅需要平衡模内色散,还需要某种机制来平衡模间色散。同时,相比单模激光器,非线性的增益和损耗(如掺杂光纤、可饱和吸收体等)都呈现出空间分布的特征,它们之间又相互影响。此外,不同的空间模式之间还存在非线性耦合效应,如模间四波混频[38,75]等。通过理解这些因素的相互作用,可以更好地设计和优化光纤锁模激光器,满足不同应用领域的需求。综上,多模光纤激光器中如何平衡色散(包括模内色散和模间色散),特别是模间色散,是实现时空耗散孤子的一个重要问题(如
3.1 时空耗散孤子中模内色散的平衡
单模光纤锁模激光器中只存在一种横模,因此只涉及模内色散。在正常色散和反常色散条件下,耗散孤子形成的机制是不同的。在反常色散腔内,克尔非线性效应和色散相互平衡,产生类似孤子的锁模[76-78];而在正常色散腔内,克尔非线性效应和色散引入的时域啁啾方向相同,因此脉冲在时域和光谱上会同时展宽。为了保持脉冲的自再现性,需要引入光谱带通滤波器来限制光谱宽度并压窄脉冲宽度[56,76,79]。类似地,多模光纤时空锁模激光器中形成时空耗散孤子的机制在正常模内色散和反常模内色散条件下可能也有所不同。时空锁模最早是在正常色散多模光纤激光器中实现的。Wright等[35]采用模间色散较小的渐变折射率多模光纤进行实验,使光在不同芯径光纤中进行耦合和光谱滤波,分别实现了空间滤波和光谱滤波,进而实现了色散(包括正常模内色散和模间色散)与腔内其他效应(如克尔非线性、空间滤波、光谱滤波、可饱和吸收体)的平衡。在这种情况下,他们成功地在1 µm 波段实现了稳定的时空耗散孤子。在随后的一段时间内,时空锁模光纤激光器的研究主要集中在正常模内色散区域[80-85]。此外,Teğin等[86]在正常色散腔中使用光栅对补偿色散实现了多模光纤色散管理腔。类似单模光纤激光器中的色散管理腔,该多模光纤激光器输出的脉冲能量得到了提升,脉冲能量大于20 nJ,压缩后的脉冲宽度为100 fs左右。
在反常色散腔中实现时空锁模同样是一个值得深入研究的问题,并且目前已经有一些关于1.5 µm和2 µm波段反常色散时空锁模的研究报道。例如,2018年,Wang等[87]成功利用半导体可饱和吸收镜(SESAM)在光纤的色度色散为负值的2 µm波段实现了时空锁模。2021年,Ma等[88]也实现了1.5 µm波段的时空锁模。2022年,Wu等[89]首次详细研究了具有反常色散的激光时空锁模问题。他们在研究中没有采用光谱滤波器,而是通过腔内的克尔非线性效应、空间滤波和可饱和吸收体来平衡色散(包括反常模内色散和模间色散),最终形成了稳定的时空耗散孤子。这些研究表明,反常色散腔中的时空耗散孤子同样具有重要的研究和应用价值。
目前,正常色散区和反常色散区都已经成功实现了时空耗散孤子,它们的实现原理可以用
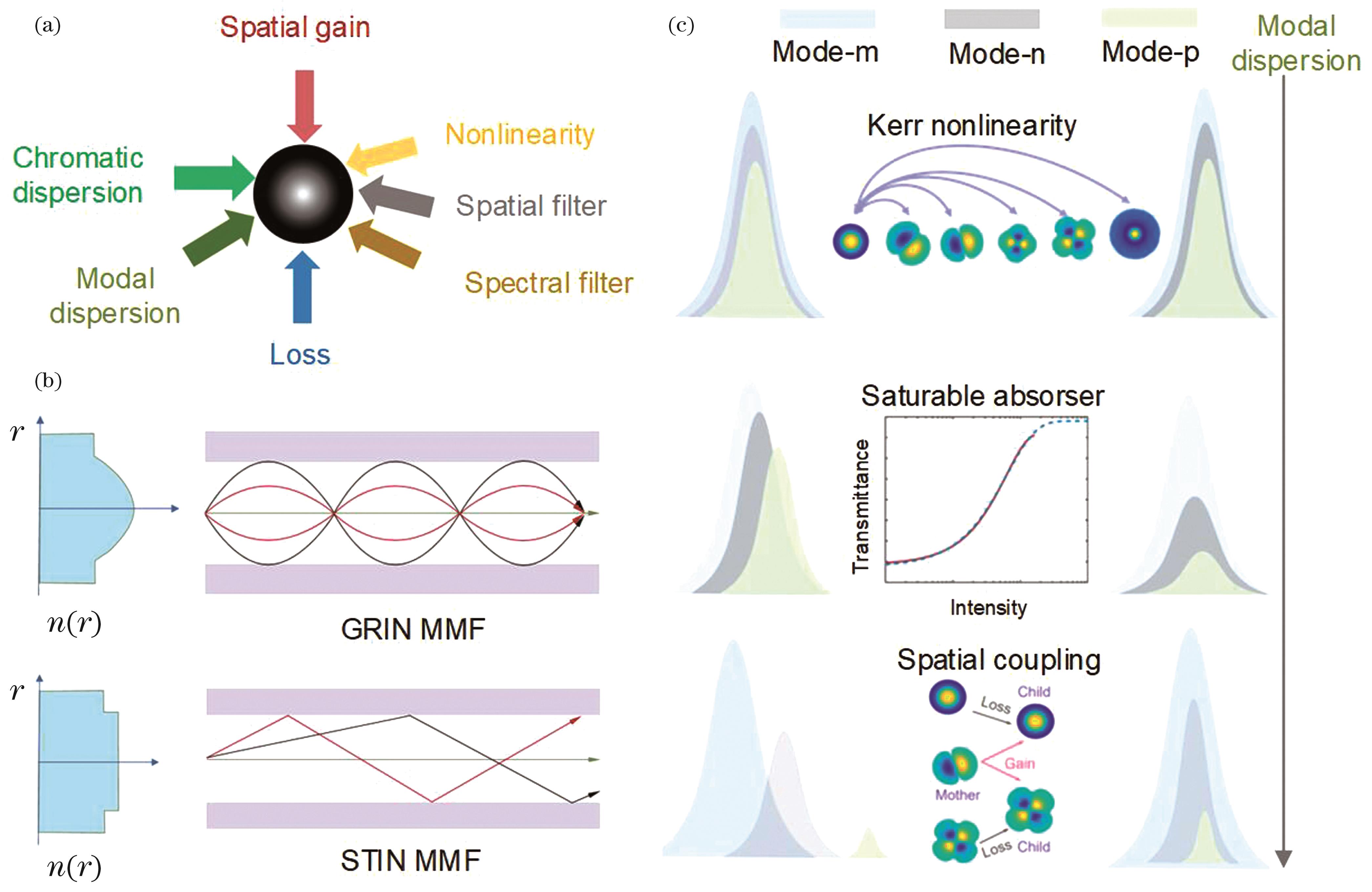
图 5. 多模光纤锁模激光器中的时空耗散孤子。(a)时空耗散孤子的物理机理,时空耗散孤子的形成依赖于平衡模间色散的腔内效应,如非线性效应和耗散效应。(b)渐变折射率光纤(上)、阶跃折射率光纤(下)的折射率分布和光线传播轨迹。(c)随着模间色散增大,平衡模间色散的三种机制不同:在弱模间色散下,不同空间模式的脉冲可以通过克尔非线性结合在一起(上);在较大模间色散下,时空可饱和吸收体可以通过饱和吸收弱脉冲来改变模式的脉冲中心,从而平衡模间走离(中);在大模间色散下,空间耦合可以衰减弱模态脉冲并使能量重新分布(从母模式到子模式),从而实现脉冲的对齐(下)
Fig. 5. Spatiotemporal dissipative solitons in multimode fiber lasers. (a) Mechanism of spatiotemporal dissipative solitons. The formation of spatiotemporal dissipative solitions relies on the intracavity effects that balance intermodal dispersion, such as nonlinear and dissipative effects. (b) Refractive index distributions and light propagation trajectories in graded-index fiber (top) and step-index fiber (bottom). (c) Schematic diagrams of pulse reactions for three mechanisms as intermode dispersion increases: Pulses for different spatial modes can bind together through Kerr nonlinearity under weak intermode dispersion (top). Pulses slightly walk off under intermediate intermode dispersion. Spatiotemporal saturable aborser can reset the walk-off by saturable absorbing weak modes and redistributing energy among modes (middle). And under large intermodal dispersion, spatial coupling can attenuate the weak mode pulses and redistribute energy from the mother-mode to the child-modes and align the modal pulse timing (bottom)
3.2 时空耗散孤子中模间色散的平衡
在多模光纤激光器中,当未对模间色散进行补偿时,不同横模在光纤中因传播速度不同将持续走离,导致激光横向分布在一个周期内无法自洽,阻碍了时空耗散孤子的形成。因此,需要通过腔内效应(如非线性和耗散效应)来平衡模间色散,从而实现时空耗散孤子。与单模光纤锁模激光器相比,多模光纤激光器中平衡空间模式之间的走离是更为关键和复杂的问题。
多模光纤可以分为渐变折射率多模光纤(GRIN MMF)和阶跃折射率多模光纤(STIN MMF),如
阶跃折射率多模光纤相对于渐变折射率多模光纤的主要优势在于其更容易制造且成本更低。此外,阶跃多模光纤通常具有更多的模式数目和更大的模态面积,这有助于实现更高的脉冲能量。因此,实现阶跃多模光纤激光器中的时空锁模有助于提高光纤激光器的输出能量,推动时空耗散孤子的实际应用。然而,阶跃多模光纤激光器目前还面临一些挑战。比如,其具有更大的模间色散和较小的非线性效应,平衡这些效应以实现时空耗散孤子相对更为复杂。因此,需要采取一些新方法(如空间滤波[93]、模式转换[94-95]等)来补偿模间色散,实现横模锁定,或者需要利用一些测量技术(如时间透镜技术[96-98]、色散傅里叶变换技术[99-101]和基于深度学习的模式分解[102-104]等)来表征多模光纤激光器的三维输出光场[40],进而有助于人们理解时空锁模的动态过程与特性。这些方法有助于优化谐振腔的结构与参数,在阶跃多模光纤激光器中实现时空锁模,从而推动这一领域的发展。
2021年,本课题组首次在包含阶跃折射率多模光纤的激光器中实现了时空耗散孤子,如
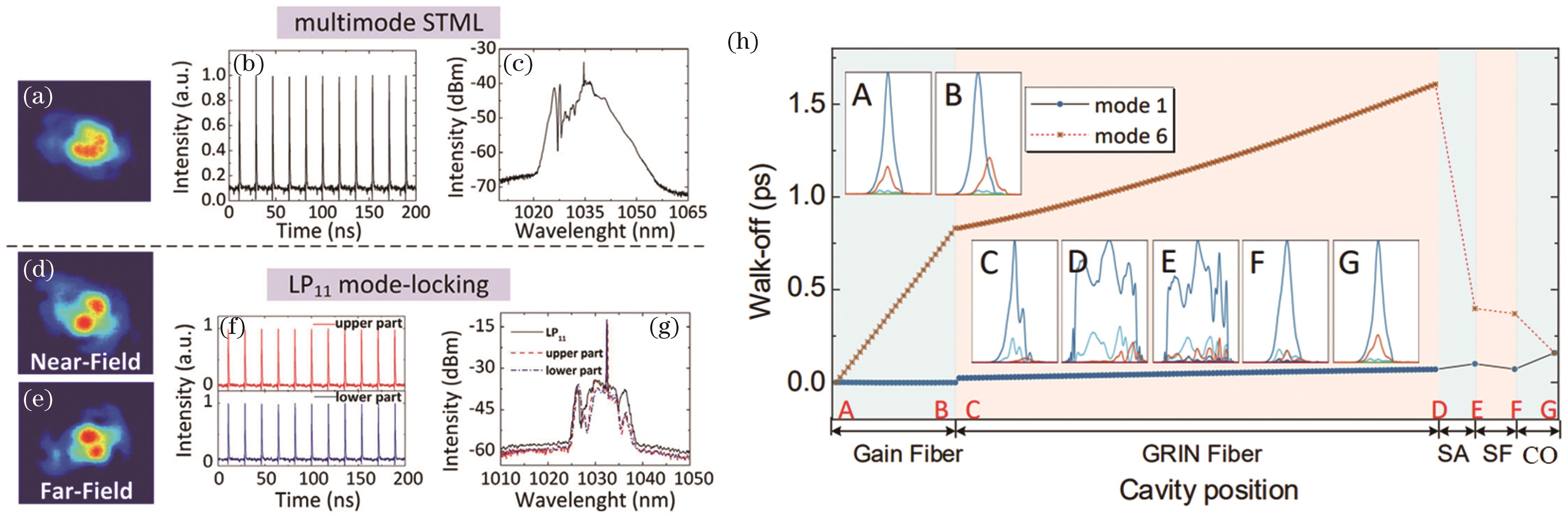
图 6. 大模间色散下的时空耗散孤子。(a)~(c)多横模锁定的光斑、时域和光谱图;(d)~(g)近单横模(LP11模式)锁模的光斑(远场和近场)、时域和光谱图;(h)锁模稳定时模式间的脉冲间距在腔内演化的仿真结果
Fig. 6. Spatiotemporal dissipative solitons with large intermode dispersion. (a)‒(c) Beam profile, pulse trance, and optical spectrum of the output pulse in the multi-transverse-mode spatiotemporal mode-locking state; (d)‒(g) beam profiles (far-field and near-field), pulse trains, and optical spectra of near-single-transverse-mode (LP11 mode) locking; (h) simulation results of the evolution of modal walk-off in the cavity
可饱和吸收体补偿模间色散的作用可以理解为:可饱和吸收体通过吸收弱光从而影响模式脉冲中心来减小模式间的走离。即:在脉冲通过可饱和吸收体之前,不同模式的走离以及不同模式脉冲的中心位置不同;可饱和吸收体的空间依赖性导致模式脉冲在不同快变时刻的损耗不同,模式脉冲的形状发生变化,从而改变脉冲中心,减小了模式之间的相对走离。值得注意的是,可饱和吸收体补偿模间走离的能力仅限于脉冲宽度范围,对于模间色散较大的激光腔,可饱和吸收体补偿色散的作用将不明显,空间耦合/滤波的作用可能更加关键。
随后,本课题组进一步探索了更大模间色散下的时空锁模,成功地在全阶跃折射率多模光纤激光器中实现了时空锁模[93]。通过调节激光腔的参数,如泵浦功率和波片位置,实现了时空锁模。如
![全阶跃折射率光纤时空锁模激光器[93]。(a)时空锁模时的输出时域和射频谱(i)、光斑(ii)、光谱以及光谱滤波结果(iii)。(b)大模间色散引起皮秒级走离的证据。(i)不同光纤长度下的输出脉冲光谱,都显示出一定的调制。(ii)由于调制作用,光谱的傅里叶变换出现了额外的峰,峰的位置一般遵循净互模色散,由实线表示。(iii)对于3.9 m长的光纤激光器,通过调整空间耦合可以观察到没有出现强光谱调制的锁模现象。(iv)输出光束轮廓。(v)输入脉冲以及在(iv)中所示的4个位置采样的脉冲的二阶自相关迹线。由于模式锁定包含可忽略的高阶模式和互模走差,迹线是平滑的。(c)仿真得到的输出光谱、光斑、一阶自相关曲线和二阶自相关曲线。(d)时空锁模时腔内不同位置模式之间的走离(这些数据是根据两种模态相对于以模态1的线性群速度运动的参考系的重心计算得到的),以及母子耦合理论图](/richHtml/zgjg/2024/51/1/0101003/img_07.jpg)
图 7. 全阶跃折射率光纤时空锁模激光器[93]。(a)时空锁模时的输出时域和射频谱(i)、光斑(ii)、光谱以及光谱滤波结果(iii)。(b)大模间色散引起皮秒级走离的证据。(i)不同光纤长度下的输出脉冲光谱,都显示出一定的调制。(ii)由于调制作用,光谱的傅里叶变换出现了额外的峰,峰的位置一般遵循净互模色散,由实线表示。(iii)对于3.9 m长的光纤激光器,通过调整空间耦合可以观察到没有出现强光谱调制的锁模现象。(iv)输出光束轮廓。(v)输入脉冲以及在(iv)中所示的4个位置采样的脉冲的二阶自相关迹线。由于模式锁定包含可忽略的高阶模式和互模走差,迹线是平滑的。(c)仿真得到的输出光谱、光斑、一阶自相关曲线和二阶自相关曲线。(d)时空锁模时腔内不同位置模式之间的走离(这些数据是根据两种模态相对于以模态1的线性群速度运动的参考系的重心计算得到的),以及母子耦合理论图
Fig. 7. All step-index fiber spatiotemporal mode-locked laser. (a) Pulse trains and radio-frequency spectra (i), beam profiles (ii), optical spectra and spectral filtering results (iii) of spatiotemporal mode-locking. (b) Evidence of picosecond of large intermode pulse walk off. (i) Optical spectra of the output pulses with different fiber lengths, all showing some modulation. (ii) As a result of the modulation, additional peaks appear in the Fourier transform of the spectra, and the positions of the peaks generally follow the net intermodal dispersion, indicated by the solid lines. (iii) For the 3.9 m long fiber laser, mode-locking without strong spectral modulation can be observed by adjusting the spatial coupling. (iv) Output beam profile for the state in panel (iii) and white curves are horizontal and vertical slices, which are Gaussian-like. (v) Second-order autocorrelation trace of the input pulse and of the pulse sampled at the four positions shown in panel (iv). Since the mode lock contains negligible higher order modes and intermodal walks, the trace is smooth. (c) Optical spectrum, beam profile, first-order autocorrelation curve, and second-order autocorrelation curve of mode-locking output pulses obtained from simulation. (d) Intracavity change of the mode-resolved pulse center position, and the right panel is an illustration of the mother-child coupling picture
为了理解模间色散是如何被补偿的,本课题组利用多模非线性薛定谔方程进行了相关仿真。仿真光斑和自相关曲线均与实验结果相似,如
时空耗散孤子的形成依赖于腔内效应(如非线性效应、耗散效应)平衡模间色散,具体的平衡机制会随着模间色散的变化而不同,如
总的来说,多模光纤激光器中时空耗散孤子的形成是一个复杂的过程,依赖于腔内多种效应的相互作用。光纤中的非线性效应用于补偿模内、模间色散;可饱和吸收体的时空可饱和吸收效应在保证时域锁模实现的同时,还可以补偿一定大小的模间色散;正常色散光纤激光器常通过加入光谱滤波来缩短高啁啾脉冲,实现自洽传播;空间滤波用于补偿模间色散,其补偿模间色散的能力比可饱和吸收体效应更强。光纤激光腔内的这些效应相互作用,可以保证增益和损耗以及色散和非线性的双重平衡,从而可以保证耗散孤子稳定存在。这些研究不仅有助于理解孤子在多模光纤激光器中的形成机制,还为光纤激光器的应用提供了新的可能性。
4 总结与展望
本文聚焦于光纤锁模激光器的耗散孤子产生机理研究,追溯了光纤锁模激光器的发展历程,回顾了光纤激光器的锁模机理:从一维时域耗散孤子锁模发展到了三维时空耗散孤子锁模。对于单模光纤锁模激光器,本文介绍了二阶色散孤子、高阶偶数色散孤子和无色散孤子的产生原理和发展;而对于多模光纤锁模激光器,本文重点介绍了模间色散的补偿原理。
近年来,出现了一些新型的光纤锁模激光器,例如Mamyshev振荡器[106-108]、基于法布里-珀罗(F-P)多模微腔的时空锁模激光器[109]等。这些新型振荡器有着不同的孤子动力学过程和输出特性,如:Mamyshev振荡器倾向于输出高能量、高峰值功率的脉冲,有望与时空锁模结合实现卓越的性能[90,110];F-P多模微腔可以得到亚飞秒时间抖动的“光子飞轮”。色散效应对这些振荡器的影响,例如对色散管理的Mamyshev振荡器、色散可设计的微型多模振荡器(F-P腔、环形腔等)的影响,还有待于进一步研究。具有不同特点和优势的振荡器有望在超快激光、光学频率梳等应用场景中发挥重要作用。
目前报道的时空锁模光纤激光器都需要进行空间滤波。如果能成功地用非线性平衡模间色散,那么空间滤波可能就不再需要了。在这种情况下,选择芯径大小相同的增益光纤和无源光纤将有望建立不需要空间滤波的全多模光纤激光器。理论上,这种无空间滤波的多模光纤激光器产生的时空耗散孤子能量将会进一步提高,有望推动时空光纤锁模激光器的应用。去除空间滤波的一个方法是补偿模间色散。单模光纤锁模激光器通过色散补偿器件对色度色散进行补偿,可以输出宽光谱的近零色散孤子、高能量的高阶偶数色散孤子和光谱平坦的无色散孤子,这些孤子都有其特征和应用场景。类似地,如果能像单模光纤激光器中补偿模内色散一样补偿多模光纤中的模间色散,势必会产生更多类型的时空耗散孤子。如果模间色散补偿为零,将不存在模式间的走离,此时锁模多模光纤激光器可能与单模光纤激光器的原理相似,但是因为其模式数目增多,故而脉冲输出能量将会更高。基于模分复用光通信的模式复用-解复用技术用于多模激光器内有望实现对模式的逐一控制,从而补偿模间色散[15]。长周期光纤光栅作为模分复用传输系统中补偿群延迟的器件,已经被用于少模光纤激光器中通过模式转换补偿模间色散,产生时空耗散孤子,但目前其模式数目不多[94]。光子灯笼[111-112]、模式转换器[95,113]、超表面[114-115]等用于模式分解和转换的器件也许可用于多模光纤激光器中实现模间色散的补偿,为实现多模光纤激光器输出更稳定、能量更高的时空耗散孤子提供了新的可能性。
综上所述,聚焦于不同色散决定的时域/时空耗散孤子,光纤锁模激光器的相关研究不仅拓展了人们对锁模原理的理解,还拓展了光纤激光器的应用领域。随着技术的不断进步以及理论的不断完善,相信光纤锁模激光器将继续发挥重要作用,并在光频梳、材料加工、医学诊断等方面实现更多应用。
[1] Diddams S A, Vahala K, Udem T. Optical frequency combs: coherently uniting the electromagnetic spectrum[J]. Science, 2020, 369(6501): eaay3676.
[2] Fermann M E, Hartl I. Ultrafast fibre lasers[J]. Nature Photonics, 2013, 7(11): 868-874.
[3] Keller U. Recent developments in compact ultrafast lasers[J]. Nature, 2003, 424(6950): 831-838.
[4] 吴修齐, 彭俊松, 张颖, 等. 智能锁模光纤激光器的原理与研究进展[J]. 中国激光, 2023, 50(11): 1101006.
[5] Hasegawa A, Tappert F. Transmission of stationary nonlinear optical pulses in dispersive dielectric fibers. II. Normal dispersion[J]. Applied Physics Letters, 1973, 23(4): 171-172.
[6] Hasegawa A, Tappert F. Transmission of stationary nonlinear optical pulses in dispersive dielectric fibers. I. Anomalous dispersion[J]. Applied Physics Letters, 1973, 23(3): 142-144.
[7] Mollenauer L F, Stolen R H, Gordon J P. Experimental observation of picosecond pulse narrowing and solitons in optical fibers[J]. Physical Review Letters, 1980, 45(13): 1095-1098.
[8] Haus H, Islam M. Theory of the soliton laser[J]. IEEE Journal of Quantum Electronics, 1985, 21(8): 1172-1188.
[9] Haus H A, Fujimoto J G, Ippen E P. Structures for additive pulse mode locking[J]. Journal of the Optical Society of America B, 1991, 8(10): 2068-2076.
[10] Kapitula T. Stability criterion for bright solitary waves of the perturbed cubic-quintic Schrödinger equation[J]. Physica D: Nonlinear Phenomena, 1998, 116(1/2): 95-120.
[11] Vanin E V, Korytin A I, Sergeev A M, et al. Dissipative optical solitons[J]. Physical Review A, 1994, 49(4): 2806-2811.
[12] Grelu P, Akhmediev N. Dissipative solitons for mode-locked lasers[J]. Nature Photonics, 2012, 6(2): 84-92.
[13] Turitsyn S K, Rosanov N N, Yarutkina I A, et al. Dissipative solitons in fiber lasers[J]. Physics-Uspekhi, 2016, 59(7): 642-668.
[14] Grelu P. Solitary waves in ultrafast fiber lasers: from solitons to dissipative solitons[J]. Optics Communications, 2024, 552: 130035.
[15] Fu W, Wright L G, Sidorenko P, et al. Several new directions for ultrafast fiber lasers[J]. Optics Express, 2018, 26(8): 9432-9463.
[16] Krupa K, Tonello A, Barthélémy A, et al. Observation of geometric parametric instability induced by the periodic spatial self-imaging of multimode waves[J]. Physical Review Letters, 2016, 116(18): 183901.
[17] Wright L G, Liu Z W, Nolan D A, et al. Self-organized instability in graded-index multimode fibres[J]. Nature Photonics, 2016, 10(12): 771-776.
[18] Teğin U, Ortaç B. Spatiotemporal instability of femtosecond pulses in graded-index multimode fibers[J]. IEEE Photonics Technology Letters, 2017, 29(24): 2195-2198.
[19] Liu Z W, Wright L G, Christodoulides D N, et al. Kerr self-cleaning of femtosecond-pulsed beams in graded-index multimode fiber[J]. Optics Letters, 2016, 41(16): 3675-3678.
[20] Krupa K, Tonello A, Shalaby B M, et al. Spatial beam self-cleaning in multimode fibres[J]. Nature Photonics, 2017, 11(4): 237-241.
[21] Niang A, Mansuryan T, Krupa K, et al. Spatial beam self-cleaning and supercontinuum generation with Yb-doped multimode graded-index fiber taper based on accelerating self-imaging and dissipative landscape[J]. Optics Express, 2019, 27(17): 24018-24028.
[22] Hasegawa A. Self-confinement of multimode optical pulse in a glass fiber[J]. Optics Letters, 1980, 5(10): 416-417.
[23] Crosignani B, di Porto P. Soliton propagation in multimode optical fibers[J]. Optics Letters, 1981, 6(7): 329-330.
[24] Renninger W H, Wise F W. Optical solitons in graded-index multimode fibres[J]. Nature Communications, 2013, 4: 1719.
[25] Ahsan A S, Agrawal G P. Graded-index solitons in multimode fibers[J]. Optics Letters, 2018, 43(14): 3345-3348.
[26] Essiambre R J, Mestre M A, Ryf R, et al. Experimental investigation of inter-modal four-wave mixing in few-mode fibers[J]. IEEE Photonics Technology Letters, 2013, 25(6): 539-542.
[27] Nazemosadat E, Pourbeyram H, Mafi A. Phase matching for spontaneous frequency conversion via four-wave mixing in graded-index multimode optical fibers[J]. Journal of the Optical Society of America B, 2016, 33(2): 144-150.
[28] Dupiol R, Bendahmane A, Krupa K, et al. Far-detuned cascaded intermodal four-wave mixing in a multimode fiber[J]. Optics Letters, 2017, 42(7): 1293-1296.
[29] Bendahmane A, Krupa K, Tonello A, et al. Seeded intermodal four-wave mixing in a highly multimode fiber[J]. Journal of the Optical Society of America B, 2018, 35(2): 295-301.
[30] Tzang O, Caravaca-Aguirre A M, Wagner K, et al. Adaptive wavefront shaping for controlling nonlinear multimode interactions in optical fibres[J]. Nature Photonics, 2018, 12(6): 368-374.
[31] Auston D. Transverse mode locking[J]. IEEE Journal of Quantum Electronics, 1968, 4(6): 420-422.
[32] Auston D. Forced and spontaneous phase locking of the transverse modes of a He-Ne laser[J]. IEEE Journal of Quantum Electronics, 1968, 4(7): 471-473.
[33] Côté D, van Driel H M. Period doubling of a femtosecond Ti∶sapphire laser by total mode locking[J]. Optics Letters, 1998, 23(9): 715-717.
[34] Wright L G, Sidorenko P, Pourbeyram H, et al. Mechanisms of spatiotemporal mode-locking[J]. Nature Physics, 2020, 16(5): 565-570.
[35] Wright L G, Christodoulides D N, Wise F W. Spatiotemporal mode-locking in multimode fiber lasers[J]. Science, 2017, 358(6359): 94-97.
[36] Richardson D J, Fini J M, Nelson L E. Space-division multiplexing in optical fibres[J]. Nature Photonics, 2013, 7(5): 354-362.
[37] Wright L G, Renninger W H, Christodoulides D N, et al. Nonlinear multimode photonics: nonlinear optics with many degrees of freedom[J]. Optica, 2022, 9(7): 824-841.
[38] Wright L G, Ziegler Z M, Lushnikov P M, et al. Multimode nonlinear fiber optics: massively parallel numerical solver, tutorial, and outlook[J]. IEEE Journal of Selected Topics in Quantum Electronics, 2018, 24(3): 5100516.
[39] Krupa K, Tonello A, Barthélémy A, et al. Multimode nonlinear fiber optics, a spatiotemporal avenue[J]. APL Photonics, 2019, 4(11): 110901.
[40] Cao B, Gao C X, Liu K W, et al. Spatiotemporal mode-locking and dissipative solitons in multimode fiber lasers[J]. Light: Science & Applications, 2023, 12: 260.
[41] 马云聪, 梁兆恒, 凌琳, 等. 智能调控多维激光技术与应用[J]. 中国激光, 2023, 50(11): 1101004.
[42] Runge A F J, Qiang Y L, Alexander T J, et al. Infinite hierarchy of solitons: interaction of Kerr nonlinearity with even orders of dispersion[J]. Physical Review Research, 2021, 3: 013166.
[43] AgrawalG P. Nonlinear fiber optics[M]//Christiansen P L, Sørensen M P, Scott A C. Nonlinear science at the dawn of the 21st century. Lecture notes in physics. Heidelberg: Springer, 2007, 542: 195-211.
[44] Richardson D J, Laming R I, Payne D N, et al. 320 fs soliton generation with passively mode-locked erbium fibre laser[J]. Electronics Letters, 1991, 27(9): 730-732.
[45] Matsas V J, Newson T P, Richardson D J, et al. Selfstarting passively mode-locked fibre ring soliton laser exploiting nonlinear polarisation rotation[J]. Electronics Letters, 1992, 28(15): 1391-1393.
[46] Wise F W, Chong A, Renninger W H. High-energy femtosecond fiber lasers based on pulse propagation at normal dispersion[J]. Laser & Photonics Reviews, 2008, 2(1/2): 58-73.
[47] KellyS M J, NoskeD U, PanditN, et al. Source of instability in fibre soliton lasers[C]∥IEE Colloquium on Fibre and Solid State Lasers, May 19, 1992, London, UK. London: IET Press, 1992.
[48] Gordon J P. Dispersive perturbations of solitons of the nonlinear Schrödinger equation[J]. Journal of the Optical Society of America B, 1992, 9(1): 91-97.
[49] Wang Q Q, Chen T, Zhang B T, et al. All-fiber passively mode-locked thulium-doped fiber ring laser using optically deposited graphene saturable absorbers[J]. Applied Physics Letters, 2013, 102(13): 131117.
[50] Tang D Y, Zhao L M, Zhao B, et al. Mechanism of multisoliton formation and soliton energy quantization in passively mode-locked fiber lasers[J]. Physical Review A, 2005, 72(4): 043816.
[51] Amrani F, Haboucha A, Salhi M, et al. Passively mode-locked erbium-doped double-clad fiber laser operating at the 322nd harmonic[J]. Optics Letters, 2009, 34(14): 2120-2122.
[52] Fermann M E, Turi L, Hofer M, et al. Additive-pulse-compression mode locking of a neodymium fiber laser[J]. Optics Letters, 1991, 16(4): 244-246.
[53] Haus H A, Tamura K, Nelson L E, et al. Stretched-pulse additive pulse mode-locking in fiber ring lasers: theory and experiment[J]. IEEE Journal of Quantum Electronics, 1995, 31(3): 591-598.
[54] Nelson L E, Jones D J, Tamura K, et al. Ultrashort-pulse fiber ring lasers[J]. Applied Physics B, 1997, 65(2): 277-294.
[55] Bélanger P A, Gagnon L, Paré C. Solitary pulses in an amplified nonlinear dispersive medium[J]. Optics Letters, 1989, 14(17): 943-945.
[56] Chong A, Buckley J, Renninger W, et al. All-normal-dispersion femtosecond fiber laser[J]. Optics Express, 2006, 14(21): 10095-10100.
[57] Kieu K, Renninger W H, Chong A, et al. Sub-100 fs pulses at watt-level powers from a dissipative-soliton fiber laser[J]. Optics Letters, 2009, 34(5): 593-595.
[58] Ortaç B, Baumgartl M, Limpert J, et al. Approaching microjoule-level pulse energy with mode-locked femtosecond fiber lasers[J]. Optics Letters, 2009, 34(10): 1585-1587.
[59] Anderson D, Desaix M, Karlsson M, et al. Wave-breaking-free pulses in nonlinear-optical fibers[J]. Journal of the Optical Society of America B, 1993, 10(7): 1185-1190.
[60] Dudley J M, Finot C, Richardson D J, et al. Self-similarity in ultrafast nonlinear optics[J]. Nature Physics, 2007, 3(9): 597-603.
[61] Ilday F Ö, Buckley J R, Clark W G, et al. Self-similar evolution of parabolic pulses in a laser[J]. Physical Review Letters, 2004, 92(21): 213902.
[62] Oktem B, Ülgüdür C, Ilday F Ö. Soliton-similariton fibre laser[J]. Nature Photonics, 2010, 4(5): 307-311.
[63] Renninger W H, Chong A, Wise F W. Self-similar pulse evolution in an all-normal-dispersion laser[J]. Physical Review A, 2010, 82(2): 021805.
[64] Elgin J N. Soliton propagation in an optical fiber with third-order dispersion[J]. Optics Letters, 1992, 17(20): 1409-1410.
[65] Dennis M L, Duling I N. Third-order dispersion in femtosecond fiber lasers[J]. Optics Letters, 1994, 19(21): 1750-1752.
[66] Blanco-Redondo A, de Sterke C M, Sipe J E, et al. Pure-quartic solitons[J]. Nature Communications, 2016, 7: 10427.
[67] Runge A F J, Hudson D D, Tam K K K, et al. The pure-quartic soliton laser[J]. Nature Photonics, 2020, 14(8): 492-497.
[68] de Sterke C M, Runge A F J, Hudson D D, et al. Pure-quartic solitons and their generalizations: theory and experiments[J]. APL Photonics, 2021, 6(9): 091101.
[69] 罗民, 张泽贤, 陈乃妙, 等. 纯四次孤子光纤激光器研究进展[J]. 物理学报, 2023, 72(20): 204203.
Luo M, Zhang Z X, Chen N M, et al. Research progress of pure quartic soliton fiber laser[J]. Acta Physica Sinica, 2023, 72(20): 204203.
[70] Liu L, Han Y, Huo , et al. Comprehensive analysis of pure-quartic soliton dynamics in a passively mode-locked fiber laser[J]. Chinese Physics B, 2023, 32(11): 114209.
[71] Karlsson M, Höök A. Soliton-like pulses governed by fourth order dispersion in optical fibers[J]. Optics Communications, 1994, 104(4/5/6): 303-307.
[72] Piché M, Cormier J F, Zhu X N. Bright optical soliton in the presence of fourth-order dispersion[J]. Optics Letters, 1996, 21(12): 845-847.
[73] Roy S, Biancalana F. Formation of quartic solitons and a localized continuum in silicon-based slot waveguides[J]. Physical Review A, 2013, 87(2): 025801.
[74] Xue X X, Grelu P, Yang B F, et al. Dispersion-less Kerr solitons in spectrally confined optical cavities[J]. Light: Science & Applications, 2023, 12: 19.
[75] HorakP, PolettiF. Multimode nonlinear fibre optics: theory and applications[M]//Yasin M, Harun S W, Arof H. Recent progress in optical fiber research. London: InTech, 2012.
[76] Song Y F, Shi X J, Wu C F, et al. Recent progress of study on optical solitons in fiber lasers[J]. Applied Physics Reviews, 2019, 6(2): 021313.
[77] Ma J, Qin Z P, Xie G Q, et al. Review of mid-infrared mode-locked laser sources in the 2.0 μm‒3.5 μm spectral region[J]. Applied Physics Reviews, 2019, 6(2): 021317.
[78] Richardson D J, Laming R I, Payne D N, et al. Selfstarting, passively modelocked erbium fibre ring laser based on the amplifying Sagnac switch[J]. Electronics Letters, 1991, 27(6): 542-544.
[79] Chong A, Renninger W H, Wise F W. All-normal-dispersion femtosecond fiber laser with pulse energy above 20 nJ[J]. Optics Letters, 2007, 32(16): 2408-2410.
[80] Qin H Q, Xiao X S, Wang P, et al. Observation of soliton molecules in a spatiotemporal mode-locked multimode fiber laser[J]. Optics Letters, 2018, 43(9): 1982-1985.
[81] Ding Y H, Xiao X S, Wang P, et al. Multiple-soliton in spatiotemporal mode-locked multimode fiber lasers[J]. Optics Express, 2019, 27(8): 11435-11446.
[82] Rigaud P, Kermene V, Simos C, et al. Dual-wavelength synchronous ultrashort pulses from a mode-locked Yb-doped multicore fiber laser with spatially dispersed gain[J]. Optics Express, 2015, 23(19): 25308-25315.
[83] Teğin U, Kakkava E, Rahmani B, et al. Spatiotemporal self-similar fiber laser[J]. Optica, 2019, 6(11): 1412-1415.
[84] Zhang H W, Lu J Y, Peng J Y, et al. Investigation of high-power spatiotemporal mode-locking with high beam quality[J]. Laser & Photonics Reviews, 2023, 17(9): 2300017.
[85] Ruan Q J, Xiao X S, Zou J H, et al. Visible-wavelength spatiotemporal mode-locked fiber laser delivering 9 ps, 4 nJ pulses at 635 nm[J]. Laser & Photonics Reviews, 2022, 16(7): 2100678.
[87] Wang Y, Tang Y L, Yan S, et al. High-power mode-locked 2 µm multimode fiber laser[J]. Laser Physics Letters, 2018, 15(8): 085101.
[88] Ma Z L, Long J G, Lin W, et al. Tunable spatiotemporal mode-locked fiber laser at 1.55 μm[J]. Optics Express, 2021, 29(6): 9465-9473.
[90] Cao B, Gao C X, Ding Y H, et al. Self-starting spatiotemporal mode-locking using Mamyshev regenerators[J]. Optics Letters, 2022, 47(17): 4584-4587.
[91] Xiao X S, Ding Y H, Fan S Z, et al. Spatiotemporal period-doubling bifurcation in mode-locked multimode fiber lasers[J]. ACS Photonics, 2022, 9(12): 3974-3980.
[92] Wright L G, Renninger W H, Christodoulides D N, et al. Spatiotemporal dynamics of multimode optical solitons[J]. Optics Express, 2015, 23(3): 3492-3506.
[93] Gao C X, Cao B, Ding Y H, et al. All-step-index-fiber spatiotemporally mode-locked laser[J]. Optica, 2023, 10(3): 356-363.
[94] Xing D K, Feng M, Liu C C, et al. Spatiotemporal mode-locked fiber laser based on dual-resonance coupling long-period fiber grating[J]. Optics Express, 2023, 31(5): 7134-7143.
[95] Wang T, Yang A, Shi F, et al. High-order mode lasing in all-FMF laser cavities[J]. Photonics Research, 2018, 7(1): 42-49.
[96] Foster M A, Salem R, Geraghty D F, et al. Silicon-chip-based ultrafast optical oscilloscope[J]. Nature, 2008, 456(7218): 81-84.
[97] Salem R, Foster M A, Gaeta A L. Application of space–time duality to ultrahigh-speed optical signal processing[J]. Advances in Optics and Photonics, 2013, 5(3): 274-317.
[98] Li B W, Huang S W, Li Y N, et al. Panoramic-reconstruction temporal imaging for seamless measurements of slowly-evolved femtosecond pulse dynamics[J]. Nature Communications, 2017, 8: 61.
[99] Guo Y K, Wen X X, Lin W, et al. Real-time multispeckle spectral-temporal measurement unveils the complexity of spatiotemporal solitons[J]. Nature Communications, 2021, 12: 67.
[102] An Y, Huang L J, Li J, et al. Deep learning-based real-time mode decomposition for multimode fibers[J]. IEEE Journal of Selected Topics in Quantum Electronics, 2020, 26(4): 4400806.
[103] An Y, Li J, Huang L J, et al. Numerical mode decomposition for multimode fiber: from multi-variable optimization to deep learning[J]. Optical Fiber Technology, 2019, 52: 101960.
[104] An Y, Huang L J, Li J, et al. Learning to decompose the modes in few-mode fibers with deep convolutional neural network[J]. Optics Express, 2019, 27(7): 10127-10137.
[105] Ding Y H, Xiao X S, Liu K W, et al. Spatiotemporal mode-locking in lasers with large modal dispersion[J]. Physical Review Letters, 2021, 126(9): 093901.
[106] Regelskis K, Želudevičius J, Viskontas K, et al. Ytterbium-doped fiber ultrashort pulse generator based on self-phase modulation and alternating spectral filtering[J]. Optics Letters, 2015, 40(22): 5255-5258.
[107] Liu Z W, Ziegler Z M, Wright L G, et al. Megawatt peak power from a Mamyshev oscillator[J]. Optica, 2017, 4(6): 649-654.
[108] Liu W, Liao R Y, Zhao J, et al. Femtosecond Mamyshev oscillator with 10-MW-level peak power[J]. Optica, 2019, 6(2): 194-197.
[109] Nie M M, Jia K P, Xie Y J, et al. Synthesized spatiotemporal mode-locking and photonic flywheel in multimode mesoresonators[J]. Nature Communications, 2022, 13: 6395.
[110] Haig H, Sidorenko P, Dhar A, et al. Multimode Mamyshev oscillator[J]. Optics Letters, 2021, 47(1): 46-49.
[111] Leon-Saval S G, Argyros A, Bland-Hawthorn J. Photonic lanterns[J]. Nanophotonics, 2013, 2(5/6): 429-440.
[112] Noordegraaf D, Skovgaard P M, Nielsen M D, et al. Efficient multi-mode to single-mode coupling in a photonic lantern[J]. Optics Express, 2009, 17(3): 1988-1994.
[113] Wang T, Wang F, Shi F, et al. Generation of femtosecond optical vortex beams in all-fiber mode-locked fiber laser using mode selective coupler[J]. Journal of Lightwave Technology, 2017, 35(11): 2161-2166.
[114] WangC S, GuiL L, QiuK Q, et al. A metasurface-assisted fiber laser enables generation of high-power and high-purity structured beams[C]∥Asia Communications and Photonics Conference/International Conference on Information Photonics and Optical Communications 2020 (ACP/IPOC), October 24-27, 2020, Beijing. Washington, DC: Optica Publishing Group, 2020: M4A.32.
Article Outline
高晨心, 曹博, 鲍成英, 杨昌喜, 肖晓晟. 光纤锁模激光器:从单模时域耗散孤子锁模到多模时空耗散孤子锁模(特邀)[J]. 中国激光, 2024, 51(1): 0101003. Chenxin Gao, Bo Cao, Chengying Bao, Changxi Yang, Xiaosheng Xiao. Mode‐Locked Fiber Lasers: from Single‐Mode Temporal Dissipative Soliton Mode‐Locking to Multimode Spatiotemporal Dissipative Soliton Mode‐Locking (Invited)[J]. Chinese Journal of Lasers, 2024, 51(1): 0101003.